Wetland ecosystems are complex natural carbon sequestration areas. These ecosystems sequester and store a relatively large amount of carbon for their areas, and understanding their function is critical to the long-term modeling of the impacts of climate change. Increasing droughts and changes in climate can alter wetlands from carbon sinks to carbon sources and can therefore create a positive feedback that could create greater emissions of long sequestered carbon back into the atmosphere. Understanding and protecting these areas is a significant part of the work that must be done to reduce the effects of human activities on the environment.
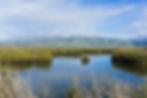
WHAT ARE WETLANDS?
Wetlands have many aliases, such as swamps, peatlands, sloughs, marshes, muskegs, bogs, fens, potholes, and mires. Wetlands exist along coasts and inland varying in size from isolated prairie potholes to vast salt marshes, in many climates and on every continent except Antarctica. Wetlands are ecotones—transition zones between dry land and water bodies. This makes wetlands neither totally dry or underwater rather they are areas of land either entirely covered by water at least part of the year or saturated with water. Often the source of water is groundwater, seeping up from an aquifer or spring but can also come from rainfall, a nearby river or lake and even the ocean, especially in coastal areas that experience strong tides.
TYPES OF NATURAL WETLANDS
There are five major types of wetlands:
marine (coastal wetlands including coastal lagoons, rocky shores, and coral reefs);
estuarine (including deltas, seagrass meadows, tidal marshes, and mangrove swamps);
lacustrine (wetlands associated with lakes);
riverine (wetlands along rivers and streams including floodplains); and
palustrine (meaning “marshy” – peatlands, marshes, swamps, and bogs).
What is the RAMSAR CONVENTION?
The Ramsar Convention (also known as the Convention on Wetlands of International Importance Especially as Waterfowl Habitat) was adopted in 1971 and is the only international convention that deals with one ecosystem or habitat type: wetlands. The Ramsar Convention was adopted in 1971 with the mission to ensure the wise use of wetlands at the local, national, and international scale. Under the Ramsar Convention, wetlands designated as Ramsar sites are sites containing representative, rare, or unique wetlands, or wetlands that are important for conserving biological diversity. In Canada there are 37 Ramsar sites spanning more than 32 million acres, one of which includes the second largest Ramsar site in the world: the Queen Maude Gulf in Nunavut. Canada is also home to the Saskatchewan River Delta which is the largest inland delta in North America and is in the process of being nominated for Ramsar designation.
WHY ARE WETLANDS IMPORTANT?
Wetlands are important for many reasons. Wetlands perform important ecological functions such as regulating water regimes, regulating global and local climates, sequestering carbon, improving water quality, offering flood mitigation and coastal protection, and providing habitat for flora and fauna (Mitra et al. 2005). Wetlands also produce goods that have a significant economic value such as fisheries, timber, peat, wildlife resources and tourism opportunities.
In terms of regulating global and local climates, wetlands play an important role in regulating exchanges of greenhouse gases (GHGs) to and from the atmosphere. These GHGs include carbon dioxide (CO2), methane (CH4), nitrous oxide (N2O), and sulfur dioxide (SO2). As part of regulating the GHG exchange, wetlands tend to be net carbon sinks for atmospheric CO2 and sources for CH4 and N2O; however, the situation varies with wetland type, spatially and temporally (Pritchard, 2009), and emissions can be highly variable. For example, coastal wetlands may have lower CH4 emission rates than freshwater wetlands as the salinity (salt content) inhibits production. Furthermore, depending on the type, wetlands can switch from carbon sinks to sources as part of a natural seasonal process or from human activities and management (Bergkamp & Orlando, 1999).
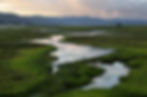
THE FUNCTION OF WETLANDS
Wetlands sequester and store carbon through photosynthesis and accumulation of organic matter in soils, sediments, and plant biomass. While many complex processes occur in wetlands, in general wetlands are carbon sinks as the wetland plants (hydrophytes)—plants that are uniquely adapted to watery (hydric) soils—grow faster than they decompose. The greater growth to decomposition rate is due to the waterlogged soils that limit oxygen diffusion into sediment profiles creating anaerobic (little to no available oxygen) conditions. These conditions also slow decomposition rates, leading to the buildup and storage of large amounts of organic carbon in wetland sediments and may produce methane.

FIGURE 1. The inland wetland carbon cycle. Carbon sequestration occurs through the accumulation of carbon absorbed by plants through photosynthesis. CO2 and CH4 emissions can occur during decomposition of organic matter. All sequestered carbon has the potential to be stored within the soil profile for centuries (Mitsch et al, 2012) unless the wetland is altered.
There are uncertainties about the overall carbon balance in wetland systems, and even about the global area of wetlands and their existing carbon stocks however it is generally agreed that wetlands comprise only about 5–8 % of the terrestrial land surface (Mitsch et al, 2012) and estimated that 20–30 % of the Earth’s soil pool of carbon (Lal 2008) is stored in wetlands (Bridgham et al. 2006). It is also
estimated that wetlands emit 20–25 % of current global methane emissions, or about 115–227 Tg CH4 per year (Whalen 2005; Bergamaschi et al. 2007; Bloom et al. 2010) and the production of N2O in undisturbed wetlands is generally low compared with terrestrial soil environments. (Page & Dalal, 2011). Once disturbed wetlands release hundreds, even thousands of years of stored carbon, into the atmosphere switching wetlands from carbon (CO2) sinks to sources.
THE THREAT TO WETLANDS
Wetlands are threatened either through drainage or desiccation. When this happens, it exposes sequestered carbon to aerobic (presence of oxygen) decomposition resulting in the emission of CO2 while simultaneously altering the natural exchange of other GHGs including CH4 and N2O (Limpert, 2020; Laine et al., 1996). The drainage or desiccation of wetlands is caused by several factors including agriculture, infrastructure development (e.g., water flow regulating devices like dams), invasion of non-native species, pollution, and climate change. For example, in southern Saskatchewan the loss of pothole wetlands continues relatively unabated and it’s been estimated by Ducks Unlimited Canada that 250,000 ha of wetlands have been lost between 1950 and 2010, and that 11.3 ha of wetlands continue to be lost each day (Yang et al., 2012).
Climate change will alter global and local hydrology which may undermine the sequestration potential of wetlands (Dudley et al, 2010). Future climate change will have two major effects on wetlands:
In most ecoregions the number of functioning wetlands (and their functional capacity) will decline and
certain wetland types will undergo a geographic shift.
According to simulations in a recent study on North American prairie wetlands, the northern short grasslands were the most vulnerable component of the prairie pothole region to temperature changes (Johnson et al., 2005). Semi-permanent wetlands in this eco-region have historically functioned on the periphery, with any increase in temperature resulting in decreasing water levels and increasing plant cover (Johnson et al. 2005).
Climate change will also impact wetlands by increasing the incidence and severity of fires and droughts; increasing salinity content; extending the range and activity of some pest and disease vectors; increasing flooding, landslide, avalanche, and mudslide damage; increased soil erosion; increasing flood runoff resulting in a decrease in recharge of some floodplain aquifers (Erwin, 2009).
WHAT ARE OUR OPTIONS?
In a world of global climate change, wetlands are considered one of the biggest unknowns of the future but are widely acknowledged as critical for climate change mitigation through capture and storage of carbon. Climate change, however, will make future efforts to restore and manage wetlands more complex especially as the different types of wetlands (e.g., floodplains, , seagrasses, saltmarshes, arctic wetlands, peatlands, and freshwater marshes)are very diverse habitats, with different stressors (Erwin, 2009). The uniqueness of each wetland means different management and restoration techniques are needed.
Appropriate responses to address climate change through wetland protection and restoration could provide “win-win” benefits in relation to other problems resulting from global warming, including benefits to biodiversity. Other benefits include maintaining other important ecological services such as provision of habitats benefitting biodiversity (e.g., fish, amphibians, and water birds) and regulation of water quantity and quality. For example, higher organic carbon levels in soils improves the moisture and nutrient holding capacities and decreases nutrient runoff into surface and groundwaters (Finlayson et al., 2011).
The diversity of wetland types and the impacts resulting from climate change requires precise projection of climate change scenarios and associated monitoring to assess how actual conditions match with the climate change models and warrants the customization of restoration remedies (Erwin, 2009). One response to the vast areas of wetlands have been impacted by agricultural conversion and drainage is restoring degraded hydric soils and ecosystems by rewetting the wetlands (Erwin, 2009) as the fluxes of GHGs in wetlands are mediated by the water level relative to the surface (Limpert, 2020). Rewetting alone is not enough; replanting is also needed as what makes wetlands effective at carbon storage is the dense above- and below-ground mats of stems and roots that trap nutrient rich debris and defend the soil against erosion or from drying (Temmink et al., 2022). The potential of GHG reduction from wetland restoration at this scale is higher than that from the rehabilitation of other types of ecosystems (Cook-Patton et al., 2020).
Apart from rewetting and replanting to maintain and improve the resiliency of wetlands so that they continue to provide important services it is important to protect remaining wetlands, maintain hydrology, reduce pollution, control non-native vegetation, and protect the biological diversity of these critical ecosystems (Erwin, 2009). A new study shown that its not too late to reverse wetland losses. Innovative research practices replicating the natural landscape-building processes—planting in large dense clumps rather than orderly equidistant rows—ensures greater restoration success as demonstrated by Temmink et al. (2022) and can enhance the carbon-storage potential of restored wetlands.
Final Thoughts
The importance of wetlands to biodiversity, climate regulation and carbon sequestration is clear. These diverse ecosystems may change rapidly in the coming decades if the impacts of climate change begin to shift the function of wetlands from carbon sinks to carbon sources releasing centuries or even millennia of stored carbon. We need to continue to explore and seek innovative opportunities to support the restoration of wetlands but with an understanding that innovative does not necessarily imply complicated or technical; the answers may already exist in nature as seen with Temmink et al. (2022). Indigenous peoples play a vital role in the protection, conservation, management, and restoration of wetlands in Canada and globally. Emerging Canadian data demonstrates that Indigenous-led nature-based solutions to climate change and biodiversity protection bring a variety of co-benefits (Vogel et al. ,2022). It is critical that we continue to protect our wetlands globally, restore the loss and degraded wetlands, and work to understand the role of wetlands in regulating our climate – especially with changing temperatures and weather patterns. Understanding the potential impact of climate change on wetlands will improve science’s ability to model future climate scenarios and guide future policy.
REFERENCES:
Bergkamp, G. & Orlando, B. (1999). Wetlands and Climate Change: Exploring collaboration between the Convention on Wetlands (Ramsar, Iran 1971) and the UN Framework Convention on Climate Change. https://www.ramsar.org/sites/default/files/documents/library/dn1999-9papere.pdf
Bergamaschi, P.C., Frankenberg, C., Meirink, J.F., Krol, M., Dentener, F., Wagner, T., Platt, U., Kaplan, J.O., Ko ̈rner, S., Heimann, M., & Goede, A. (2007). Satellite cartography of atmospheric methane from SCIAMACHY on board ENVISAT: 2. Evaluation based on inverse model simulations. J Geophys Res 112: D02304. doi:10.1029/2006JD007268
Bloom, A.A., Palmer, P.I., Fraser, A., Reay, D.S., & Frankenberg, C. (2010). Large-scale controls of methanogenesis inferred from methane and gravity spaceborne data. Science 327:322–325
Bridgham SD, Megonigal JP, Keller JK, Bliss NB, Trettin C (2006) The carbon balance of North American wetlands. Wetlands 26:889–916
Briggs J., Large, D.J., Snape, C., Drage, T., Whittles, D., Cooper, M., Macquaker, J.H.S., & Spiro, B.F. (2007). Influence of climate and hydrology on carbon in an early Miocene peatland. Earth Planet Sci Lett 253:445–454
Cook-Patton, S. C. et al. (2020) Mapping carbon accumulation potential from global natural forest regrowth. Nature 585, 545–550.
Dudley, N, Stolton, S, Belokurov, A, Krueger, L, Lopoukhine, N, McaKinnon, K, Sandwith, T, & Kekhran, N (eds). (2010). Natural Solutions: Protected areas helping people cope with climate change, A report funded and commissioned by IUCN-WCPA, TNC, UNDP, WCS, The World Bank and WWF, Gland, Sitzerland, Washington DC, and New York, USA. https://www.iucn.org/content/natural-solutions-protected-areas-helping-people-cope-climate-change
Erwin, K. (2009). Wetlands and Global Climate Change: The Role of Wetland Restoration in a Changing World. Wetlands Ecology and Management. 17. 71-84. 10.1007/s11273-008-9119-1.
Finlayson, C.M., Davis, J.A., Gell, P.A., Kingsford, R.T. & Parton, K.A. (2011). The status of wetlands and the predicted effects of global climate change: the situation in Australia, Aquatic Sciences, published online Springer Basel
Johnson, Carter W.; Millett, B. V.; Gilmanov, T.; Guntenspergen, G. R.; and Naugle, David E., "Vulnerability of Northern Prairie Wetlands to Climate Change" (2005). Wildlife Biology Faculty Publications. 46. https://scholarworks.umt.edu/wildbio_pubs/46
Koch, M.S., Schopmeyer, S.A., Kyhn-Hansen, C., Madden, C.J., & Peters, J.S. (2007). Tropical seagrass species tolerance to hypersalinity stress. Aquat Bot 86:14–24
Lal, R. (2008). Carbon sequestration. Philosophical transactions of the Royal Society of London. Series B, Biological sciences. 363. 815-30. 10.1098/rstb.2007.2185.
Laine, J. et al. (1996) Effect of water-level drawdown on global climatic warming: northern peatlands. AMBIO 25, 179–184.
Limpert, K. E. et al. Reducing emissions from degraded floodplain wetlands. Front. Environ. Sci. 8, 8 (2020); https://doi.org/10.3389/fenvs.2020.00008
Mitra, S., Wassmann, R., & Vlek, P.L.G. (2005). An appraisal of global wetland area and its organic carbon stock. Curr Sci 88:25–35
Mitsch, W., Bernal, B., Nahlik, A., Mander, Ü., Zhang, L., Anderson, C., Jørgensen, S.E. & Brix, Hans. (2012). Wetlands, carbon, and climate change. Landscape Ecology. 28. 10.1007/s10980-012-9758-8.
Mote, P.W., Parson, E.A., Hamlet, A.F., Keeton, W.S., Lettenmaier, D., Mantua, N., Miles, E.L., Peterson, D.W., Peterson, D.L., Slaughter, R., Snover, A.K. (2003). Preparing for climatic change: the water, salmon, and forests of the Pacific Northwest. Clim Change 61:45–88
Page, S.E., & Baird, A.J. (2016). Peatlands and global change: response and resilience. Annu. Rev. Environ. Resour., 41 (2016), pp. 35-57
Page, K. & Dalal, R. (2011). Contribution of natural and drained wetland systems to carbon stocks, CO2, N2O, and CH4 fluxes: An Australian perspective. Soil Research. 49. 377-388. 10.1071/SR11024.
Pritchard, D. (2009). Reducing Emissions from Deforestation and Forest Degradation in developing countries. https://www.eldis.org/document/A42802
Ramsar Convention Secretariat, 2013. The Ramsar Convention Manual: a guide to the Convention on Wetlands (Ramsar, Iran, 1971), 6th ed. Ramsar Convention Secretariat, Gland, Switzerland. https://www.ramsar.org/document/ramsar-convention-manual-6th-edition
Short, F.T. & Neckles, H.A. (1999). The effects of global climate change on seagrasses. Aquat Bot 65:83–96. 10.1016/S0304-3770(98)00117-X.
Temmink, R.J.M, Lamers, L.P.M., Angelini, C., Bouma, T.J., Fritz, C., van de Koppel, J., Lexmond, R., Rietkerk, M., Silliman, B.R., Joosten, H., & van der Heide, T. (2022). Recovering wetland biogeomorphic feedbacks to restore the world’s biotic carbon hotspots. Science; 376 (6593) DOI: 10.1126/science.abn1479
Vogel, B., Yumagulova, L., McBean, G., & Charles Norris, K. A. (2022). Indigenous-Led Nature-Based Solutions for the Climate Crisis: Insights from Canada. Sustainability, 14(11), 6725. MDPI AG. Retrieved from http://dx.doi.org/10.3390/su14116725
Walker, D.I. (1985). Correlations between salinity and the growth of the seagrass Amphibolis Antarctica (Labill. Sonders and Aschers., in Shark Bay, Western Australia, using a new method for measuring production rate. Aquat Bot 23:13–26
Were, D., Kansiime, F., Fetahi, T., Cooper, A., & Jjuuko, C. (2019). Carbon Sequestration by Wetlands: A Critical Review of Enhancement Measures for Climate Change Mitigation. Earth Systems and Environment. 3. 10.1007/s41748-019-00094-0.
Whalen, S.C. (2005). Biogeochemistry of methane exchange between natural wetlands and the atmosphere. Environ Eng Sci 22:73–94
Yang, W., Liu, Y., Ou, C., & Gabor, S. (2016). Examining water quality effects of riparian wetland loss and restoration scenarios in a southern Ontario water shed J. Environ. Manag., 174, pp. 26-34, https://doi.org/10.1016/j.jenvman.2016.03.001
Contributors
Researchers
Katarina Duke
Mauro Aiello, Ph.D.
Lead Author
Katarina Duke
Lark Scientific Financial Support
Axel Doerwald
Graphics
Adri Poggetti